Summer 2022 - Vol. 17, No. 1
SCIENTIFIC REPORT
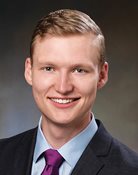
Tesman Lau
Two Cases of Syncope in Children
Garret Tesman, MD
Kyle Lau, MD
Family Physicians
Family Medicine Residency Program
Penn Medicine Lancaster General Health
Emmanuelle Favilla, MD
Pediatric Cardiologist
CHOP Specialty Care
Syncope is an acute, transient loss of consciousness associated with loss of postural tone and spontaneous recovery. Syncope is a common problem in children presenting to emergency departments. Before adolescence, about 15% of children have at least one episode. The common causes include vasovagal reactions, orthostatic reactions, breath-holding spells, and reactions to acute ingestions. Only 2% of patients have a serious underlying cardiac cause.
1 While less common, it’s important to rule out insidious cardiac causes.
As is the case with all presenting chief complaints, a thorough family history is helpful in elucidating the cause. Regarding posture, timing of the event and the associated activity are important. Pallor, diaphoresis, visual or auditory changes, dizziness, palpitations, hyperventilation, nausea, incontinence, seizure-like activity (including timing of seizures), presence of a postictal state, and prodromal vision/hearing changes can be helpful. The absence of vigorous exercise or pain, and a history of skipped meals, point toward a non-cardiac cause. Exertional syncope is a good indicator that the etiology may be cardiac.
Children with mid-exertional syncope may be manifesting cardiac disease and warrant evaluation.
2 One also must be vigilant for red flags associated with events occurring during periods of high emotions, as they relate to potential catecholamine surges.
Physical examination for the undifferentiated syncopal event should include orthostatic vitals. Auscultation of murmurs is helpful, especially those consistent with left ventricular outflow tract disease and mitral regurgitation. Characterizing a particularly prominent S2 is also important, as it may indicate pulmonary hypertension.
3
The cardiac etiologies of syncope can be grouped by the following general framework: electrical, structural, or acquired. The differential for each is broad. See Fig. 1 for common causes and their incidence.
Some important electrical etiologies to consider include Wolff-Parkinson-White (WPW), Long QT syndrome, Brugada syndrome, catecholaminergic polymorphic ventricular tachycardia (CPVT), and arrhythmogenic right ventricular cardiomyopathy (ARVC). This review will focus on only a few electrical and structural syndromes.
3
CASE 1
An otherwise healthy 12-year-old male presents to the office for pre-participation school sports screening. His mother reports one episode of syncope during a particularly hot summer day while playing soccer, which they had attributed to dehydration. On further questioning, he states that he sometimes has “a funny feeling in his chest” during other sports. There is no family history of cardiac disorders.
An EKG (see Fig. 2) reveals a shortened PR interval and a wide QRS complex with a slurred onset of the QRS waveform, consistent with the WPW pattern. How would you counsel the patient and his parents regarding this finding, and is a cardiology consult warranted?
Fig. 2. EKG showing shortened PR interval and wide QRS complex.3
Pathophysiology
In the normal heart, electrical impulses travel from the atria and are delayed by the AV node before moving on to the ventricles. WPW is characterized by an accessory pathway, also known as the bundle of Kent, that bypasses all or part of the AV node. This additional conduction tissue is congenital and is the result of remnant myocardial syncytium at the annulus fibrosus that failed to resorb during fetal development. It is more conductive, and as electrical impulses take the path of least resistance, we see a preexcitation of the ventricles.
4
When conduction bypasses the AV node, we see a shortening of the PR interval; in the case of WPW, we see a characteristic “slurred” upstroke of the QRS complex known as a delta wave. The WPW pattern on the EKG may be intermittent and may even disappear permanently over time. In several large cohorts, the frequency of intermittent preexcitation appears to range from 10% to 40%.
5
This accessory pathway does not lead to sudden events; however, it is a nidus for re-entrant SVTs both in children and adults. In the adult population — and rarely pediatric patients — should atrial fibrillation occur, the accessory pathway enables the arrhythmia to deteriorate to ventricular fibrillation (VF).
Wolff-Parkinson-White Epidemiology
In a review of more than 22,000 adults, the prevalence of a WPW pattern on EKG is estimated to be between 0.13% and 0.25% of the general population. Only 1.8% of those with the preexcitation pattern had documented arrhythmias, i.e., WPW syndrome. In a study of over 430,000 children, ages 6 to 20 years, the prevalence of WPW syndrome was 0.07%. Males are more commonly affected than females. A proportion of WPW cases are due to a familial syndrome, but many have no family history of this disorder.
WPW EKG Pattern
The WPW EKG pattern has two classic components: first, a PR interval less than 0.12 seconds due to rapid conduction of the accessory pathway that bypasses the AV node; and second, slurring of the upstroke of QRS complex. It should be noted that this classic pattern occurs only when the accessory pathway conducts in an antegrade direction. If the pathway conducts retrograde, from the ventricles to the atria, there will be no delta wave.
4
Due to the electrophysiological changes of WPW, EKG findings can be similar to those seen in myocardial infarction (MI), premature ventricular contractions (PVCs), cardiomyopathies, and bundle branch blocks. A negative delta wave may mimic a Q wave, and a positive delta wave may obscure one. Intermittent WPW is occasionally seen on alternate beats and may resemble ventricular bigeminy. If the pattern persists for several beats, it can resemble an accelerated idioventricular rhythm. Preexcited beats are sometimes confused with left or right bundle branch block. EKG changes due to congenital heart defects such as atrial enlargement or hypertrophic cardiomyopathy can sometimes be confused with WPW due to abnormal depolarization.
4
Workup and Risk Stratification
There are differing opinions on the approach to patients with preexcitation, but there is consensus that adolescent/pediatric patients discovered to have this rhythm should be referred to a specialist in pediatric cardiology/electrophysiology (EP) to initiate risk stratification. Typically, this includes exercise testing to determine the shortest cardiac cycle length at which the accessory pathway is suppressed, if at all, and potentially requires invasive electrophysiology studies.
6
Management
With referral, treatment, and patient education, patients with WPW syndrome can expect to have a normal life expectancy and good quality of life.
7 Patients with WPW syndrome may be treated acutely with adenosine. Caution should be used in the use of AV nodal blocking agents, as these can propagate deterioration of the arrhythmia, should the patient be in atrial fibrillation and not re-entrant SVT. Radiofrequency ablation is first-line treatment for those with symptomatic tachyarrhythmias and those deemed higher risk based on EP studies or cardiac history. Unstable patients require immediate cardioversion.
8
Management of asymptomatic individuals is less clear given the low risk of arrhythmia and sudden death, which appeared comparable to the risk of ablation in a recent meta-analysis.
9 If episodes of SVT are infrequent but last more than one hour, a “pill-in-the-pocket” approach may be effective. These patients can self-administer an as-needed dose of non-dihydropyridine calcium channel blockers, beta blockers, or antiarrhythmics. Effectiveness of this approach ranges from 30% to 60%.
10 Shared decision-making with input from cardiology is important in deciding what plan is most appropriate.
Summary
The WPW syndrome is due to an accessory pathway of the AV ring and causes a pattern on EKG classically described as a shortened PR interval with a slurred upstroke of the QRS complex, termed a delta wave. This pattern may be intermittent. The prevalence of the WPW pattern is estimated to be 0.13% to 0.25% and may disappear permanently over time. Most asymptomatic patients can be cautiously observed; a cardiology referral for our patient regarding risk stratification yielded this advice.
CASE 2
A 13-year-old female presented after “passing out” during gym class. She was found to be pulseless, thus her coach quickly applied an AED and gave one shock before she was transferred to the hospital where further evaluation was performed. An EKG showed left ventricular hypertrophy and demonstrated Q waves in the inferior and lateral leads. An echocardiogram confirmed left ventricular hypertrophy. The patient was diagnosed with hypertrophic cardiomyopathy.
Epidemiology of Pediatric Hypertrophic Cardiomyopathy
The incidence of cardiomyopathy in children younger than 18 years old is 0.47 to 1.5 cases per 100,000 per year.
11,12 Hypertrophic cardiomyopathy (HCM) is the most commonly inherited cardiomyopathy and can be caused by various genetic mutations. Pediatric HCM incorporates a large group of various disorders. Primary HCM is caused by a sarcomeric gene mutation and is the most common cause. Secondary HCM, or non-sarcomeric-caused HCM, includes inborn errors of metabolism, malformation syndrome, neuromuscular disease, and mitochondrial disease, which are responsible for approximately 35% of HCM in children worldwide.
Inborn errors of metabolism and malformation syndromes are common causes of HCM in infancy, and are often associated with neurological and musculoskeletal abnormalities.
12 In contrast, HCM due to sarcomeric mutations may not be diagnosed until adolescence or early adulthood, and are most commonly those identified as “familial cases.” Therefore, age at presentation and the HCM etiology are important for predicting outcomes and progression of the disease.
13
Pediatric Hypertrophic Cardiomyopathy Pathophysiology
HCM is characterized by hypertrophy of cardiac myocytes without dilatation of the left ventricle (LV), and most commonly, normal left ventricular systolic function. The phenotype familiar to most primary care practitioners is that of hypertrophy of the basal portion of the septum causing left ventricular outflow tract (LVOT) obstruction in the setting of concurrent systolic anterior motion of the mitral valve. However, it is important to remain vigilant regarding subtle cases that involve other areas of the myocardium, including solely the apex. Cardiac myocytes are not only hypertrophied but also disorganized and separated by areas of fibrosis which can lead to arrhythmias.
14
Arrhythmias and a primary hemodynamic mechanism in the setting of LVOT obstruction are the two underlying mechanisms for syncope in patients with HCM. Prolonged repolarization of transmembrane action potentials and changes of composition and ion channels in hypertrophic cardiac myocytes can also cause arrhythmias. Finally, repetitive microvascular ischemia can cause scarring, further precipitating ventricular tachyarrhythmias and atrial fibrillation.
14
Clinical Presentation
Patients with HCM can have a wide range of presentations, from remaining asymptomatic to having palpitations, chest pain, cardiac arrest, and even sudden death.
Hypertrophied cardiomyocytes with an increased oxygen requirement may become ischemic because the thickened heart wall reduces blood flow by narrowing the lumen of coronary arteries. Patients may complain of exertional chest pain that improves with rest. LVOT obstruction may also lead to palpitations, exertional pre-syncope, or syncope. Palpitations in these patients need to be thoroughly evaluated as they can represent both atrial and ventricular ectopy/ tachyarrhythmias.
Sudden cardiac death (SCD) is mostly caused by deterioration of a ventricular arrhythmia. Atrial fibrillation (AF) can be found in about 25% of HCM and LVOT obstruction patients. Dilation of the left atrium and LVOT obstruction are common causes of AF. In turn, AF may lead to heart failure, left ventricular end diastolic pressure elevation, and thromboemboli.
13
On physical examination, patients with HCM may demonstrate signs of left ventricular hypertrophy (LVH), such as leftward cardiac impulse displacement, cannon A wave, prominent S4, or presystolic apical lift. The LVOT obstruction causes a murmur characterized as a harsh and mid-systolic heartbeat at the apex and left sternal border. Maneuvers that decrease LV volume will increase the intensity of the murmur. In addition, a mitral valve regurgitation murmur can be common in patients with HCM.
14
Workup
Evaluation of suspected HCM starts with an EKG to look for pathologic Q waves and abnormal repolarizations. Signs of LVH on EKG are nonspecific and found in normal children and adolescents. While cardiac MRI is considered the gold standard for diagnosis, an echocardiogram is 80% specific.
15,16 Regarding echocardiogram, LV wall thickness greater than 15 mm that is not explained by other factors is considered diagnostic. Other echocardiogram findings suggestive of HCM include LVOT obstruction, especially in the setting of systolic anterior motion of the mitral valve leaflet.
Patients with metabolic HCM may have an echocardiogram that shows a concentric biventricular hypertrophy. In contrast, sarcomeric and syndromic HCM echocardiograms tend to have an asymmetrical septal hypertrophy. LVOT obstruction is seen in 25% to 40% of children with HCM. Diastolic dysfunction as detected by echo can be an early indication of the development of HCM, as can the presence of Q waves on EKG or arrhythmias in those at risk of developing it.
14
All patients with HCM should be offered genetic testing and counseling, and if a pathogenic variant is the cause, genetic testing should be offered to all first-degree relatives as well. Sarcomeric HCM is inherited in an autosomal dominant pattern with variable expression and incomplete penetrance. First-degree relatives found to be positive for the pathogenic genotype should be offered screening imaging and ongoing clinical surveillance.
14,17,18
If a patient does not have pathologic HCM genetic variants, then first-degree relatives do not need genetic testing. However, genetic screening is evolving, thus the discussion of whether to test may be an ongoing conversation.
14
Patients should also be screened for SCD risk factors, including previous adverse cardiac events, non-sustained ventricular tachycardia, unexplained syncope, family history of early HCM-related SCD, and extreme LVH.
16 According to the 2020 American Heart Association guidelines, further risk factors such as apical aneurysm, decreased LV systolic function, and extensive gadolinium enhancement should be considered.
Treatment
Patients should be referred to pediatric cardiology after being diagnosed with HCM or if there is high clinical suspicion. Cardiovascular magnetic resonance (CMR) may be useful in patients with inconclusive echocardiogram results, especially if suspected of having metabolic storage disorder. CMR may also assist with assessing the risk of SCD by measuring late gadolinium enhancement.
19
ICD implant should be considered for any patient with SCD risks factors, and patients with HCM and a previous documented cardiac arrest or sustained ventricular tachycardia should consider an ICD for secondary prevention of SCD.
20
Symptom control and prevention of SCD are the main goals of treatment. Symptomatic treatment of adult HCM is well established, however pediatric HCM is not well studied. The underlying approach is the same:
- avoid peripheral vasodilatation,
- maintain adequate intravascular volume, and
- consider beta blockers to allow increased filling time in those with severe hypertrophy or obstruction.
Beta blockers have also been shown to prevent SCD events. If beta blockers are contraindicated or do not improve symptoms, then a non-dihydropyridine calcium channel blocker like verapamil or diltiazem can be added. Disopyramide can be considered for patients with persistent symptoms. Diuretics should be avoided as they can deplete intravascular volume.
21
An anticoagulant should be considered for stroke prevention if patients have atrial arrhythmias. Patients with AF with rapid ventricular rate (RVR) may benefit from medications that control either rate or rhythm.
21
Septal reduction can be done by surgery or ablation. The goal of this procedure is to reduce LVOT obstruction. Mitral valve regurgitation may improve after septal myectomy, but valves can be injured during the surgery.
22 Treatment for those with severe cases can also include cardiac resynchronization, LV assistant device, or cardiac transplantation.
21
Advice regarding exercise for children with HCM is a controversial topic. Exercise restriction for adolescents can affect their mental health and lead to social isolation and depression. Most recent information suggests that moderate-intensity exercise could be useful and does not increase the risk of arrhythmias.
22 Higher intensity exercise may be pursued if they are genotype-positive, but phenotype-negative. Most importantly, shared decision-making and multidisciplinary care are essential and can lead to better outcomes.
23
Summary
Like all patients, pediatric patients with syncope should be evaluated for possible cardiac causes. Syncope with exertion, or soon after, is concerning, as HCM can result in LVOT obstruction or arrhythmias. An EKG and echocardiogram should be done in patients suspected of having cardiac etiology.
Returning to the patient described in the second case, initial management of HCM includes removing stressors, ensuring adequate hydration, and avoiding peripheral vasodilatation to limit symptoms. Restricting activity until further evaluation is prudent; screening and surveillance of her first-degree relatives is recommended. A referral to pediatric cardiology will assist in management, as will shared decision-making and a frank conversation about the value of exercises and the balance of lifetime risk factors.
REFERENCES
1. Miyake, Christina Y., et al. Risk of cardiac disease and observations on lack of potential predictors by clinical history among children presenting for cardiac evaluation of mid-exertional syncope.
Cardiology in the Young. 2015;26;894–900.
2. Hurst D, Hirsh D, Oster ME, et al. Syncope in the pediatric emergency department — can we predict cardiac disease based on history alone?
J Emerg Med. 2015;49:1-7.
3. Favilla, Emmanuelle. “Syncope in Children.” Lecture, Lancaster General Hospital, September 18, 2021.
4. Wang K, Asinger R, Hodges M. Electrocardiograms of Wolff-Parkinson-White syndrome simulating other conditions.
Am Heart J. 1996;132:152.
5. Kiger ME, McCanta AC, Tong S, et al. Intermittent versus persistent Wolff-Parkinson-White syndrome in children: electrophysiologic properties and clinical outcomes.
Pacing Clin Electrophysiol. 2016;39:14.
6. Sternick EB. Familial pseudo-WPW syndrome.
J Cardiovasc Electrophysio. 2009;20:E62.
7. Al-Khatib SM, Arshad A, Balk EM, et al. Risk stratification for arrhythmic events in patients with asymptomatic pre-excitation: a systematic review for the 2015 ACC/AHA/HRS Guideline for the Management of Adult Patients with Supraventricular Tachycardia: a report of the American College of Cardiology/American Heart Association Task Force on Clinical Practice Guidelines and the Heart Rhythm Society.
Circulation. 2016;133:e575.
8. Helton M. Diagnosis and management of common types of supraventricular tachycardia.
Am Fam Physician. 2015;92(9):793-800.
9. Obeyesekere M, Klein G. The asymptomatic Wolff-Parkinson-White patient: time to be more proactive?
Circulation. 2014;130(10):805–807.
10. Alboni P, Tomasi C, Menozzi C, et al. Efficacy and safety of out-of-hospital self-administered single-dose oral drug treatment in the management of infrequent, well-tolerated paroxysmal supraventricular tachycardia.
J Am Coll Cardiol. 2001;37(2):548-553.
11. Lee T, Hsu D, Kantor P, et al. Pediatric cardiomyopathies.
Circ Res. 2017;121(7):855-873.
12. Colan SD, Lipshultz SE, Lowe AM, et al. Epidemiology and cause-specific outcome of hypertrophic cardiomyopathy in children.
Circulation. 2007;115(6):773-781.
13. Marian AJ, Braunwald E. Hypertrophic cardiomyopathy: genetics, pathogenesis, clinical manifestations, diagnosis, and therapy.
Circ Res. 2017;121(7):749-770.
14. Kantor PF, Casarez TW, Bar-Cohen Y. Hypertrophic cardiomyopathy in adolescence.
J Am Coll Cardiol Case Rep. 2021;3(1):10-15.
15. Czimbalmos C, Csecs I, Toth A, et al. The demanding grey zone: sport indices by cardiac magnetic resonance imaging differentiate hypertrophic cardiomyopathy from athlete's heart.
PLoS One. 2019;14(2):e0211624.
16. Raj MA, Ranka S, Goyal A. Hypertrophic obstructive cardiomyopathy. In:
StatPearls [Internet]. StatPearls Publishing: 2022. Accessed April 27, 2022.
https://www.ncbi.nlm.nih.gov/books/NBK430820/
17. Mital S. A pediatric perspective on the ACC/AHA hypertrophic cardiomyopathy guidelines. American College of Cardiology 2021. Available at
https://www.acc.org/latest-in-cardiology/articles/2021/04/01/13/02/a-pediatric-perspective-on-the-acc-aha-hypertrophic-cardiomyopathy-guidelines. Accessed April 27, 2022.
18. Alfares AA, Kelly MA, McDermott G, et al. Results of clinical genetic testing of 2,912 probands with hypertrophic cardiomyopathy: expanded panels offer limited additional sensitivity [published correction appears in
Genet Med. 2015 Apr;17(4):319]. Genet Med. 2015;17(11):880-888.
19. Windram JD, Dragelescu A, Benson L. Myocardial dimensions in children with hypertrophic cardiomyopathy: a comparison between echocardiography and cardiac magnetic resonance imaging.
Can J Cardiol. 2016;32(12):1507-1512.
20. Decker JA, Rossano JW, Smith EO, et al. Risk factors and mode of death in isolated hypertrophic cardiomyopathy in children.
J Am Coll Cardiol. 2009;54(3):250-254.
21. Ommen SR, Mital S, Burke MA, et al. 2020 AHA/ACC guideline for the diagnosis and treatment of patients with hypertrophic cardiomyopathy.
Circulation. 2020;142(25):e558-e631.
22. Altarabsheh SE, Dearani JA, Burkhart HM, et al. Outcome of septal myectomy for obstructive hypertrophic cardiomyopathy in children and young adults.
Ann Thorac Surg. 2013;95(2):663-669.
23. O’Mahony C, Jichi F, Pavlou M. A novel clinical risk prediction model for sudden cardiac death in hypertrophic cardiomyopathy (HCM Risk-SCD).
Eur Heart J. 2014;35(30):2010-2020.